Contents
- 1 循環の神経制御 (Nervous Regulation of the Circulation)
- 2 自律神経系 (Autonomic Nervous System)
- 3 交感神経血管収縮系と中枢神経系によるその制御 (Sympathetic Vasoconstrictor System and Its Control by the Central Nervous System)
- 4 血管の持続的な部分的収縮—交感神経血管収縮トーン (Continuous Partial Constriction of Blood Vessels by Sympathetic Vasoconstrictor Tone)
- 5 血管運動中枢による心臓活動の制御 (Control of Heart Activity by the Vasomotor Center)
- 6 高位神経中枢による血管運動中枢の制御 (Control of the Vasomotor Center by Higher Nervous Centers)
- 7 交感神経血管収縮神経伝達物質—ノルエピネフリン (Norepinephrine Is the Sympathetic Vasoconstrictor Neurotransmitter)
- 8 副腎髄質と交感神経血管収縮系との関係 (Adrenal Medullae and Their Relationship to the Sympathetic Vasoconstrictor System)
- 9 交感神経血管拡張系とその中枢神経系による制御 (Sympathetic Vasodilator System and Its Control by the Central Nervous System)
- 10 交感神経血管拡張系の役割 (Possible Role of the Sympathetic Vasodilator System)
- 11 感情的失神—血管迷走神経性失神 (Emotional Fainting—Vasovagal Syncope)
- 12 動脈圧の迅速な制御における神経系の役割 (Role of the Nervous System in Rapid Control of Arterial Pressure)
- 13 動脈圧の神経制御の迅速さ (Nervous Control of Arterial Pressure Is Rapid)
- 14 筋肉運動および他のストレス時の動脈圧の上昇 (Increases in Arterial Pressure During Muscle Exercise and Other Stresses)
- 15 動脈圧を正常に保つための反射機構 (Reflex Mechanisms for Maintaining Normal Arterial Pressure)
- 16 バロレセプター動脈圧制御システム—バロレセプター反射 (Baroreceptor Arterial Pressure Control System—Baroreceptor Reflexes) バロ:圧
- 17 バロレセプターによって引き起こされる循環反射 (Circulatory Reflex Initiated by the Baroreceptors)
- 18 長期的な動脈圧調整におけるバロレセプターの重要性 (Are the Baroreceptors Important in Long-Term Regulation of Arterial Pressure?)
- 19 頸動脈および大動脈の化学受容体による動脈圧の制御—低酸素の動脈圧への影響 (Control of Arterial Pressure by the Carotid and Aortic Chemoreceptors—Effect of Low Oxygen on Arterial Pressure)
- 20 化学受容器による動脈圧制御のメカニズム (Mechanism of Arterial Pressure Control by Chemoreceptors)
- 21 心房および肺動脈反射による動脈圧の調整 (Atrial and Pulmonary Artery Reflexes Regulate Arterial Pressure)
- 22 心房反射による腎臓の活性化—容積反射 (Atrial Reflexes That Activate the Kidneys—The Volume Reflex)
- 23 心房圧の増加による心拍数の増加—ベインブリッジ反射 (Increased Atrial Pressure Raises Heart Rate—Bainbridge Reflex)
- 24 脳血管運動中枢への血流減少による動脈圧の上昇—中枢神経系虚血反応 (Decreased Blood Flow to Brain Vasomotor Center Elicits Increased Blood Pressure—CNS Ischemic Response)
- 25 動脈圧の神経制御における特殊な特徴 (Special Features of Nervous Control of Arterial Pressure)
- 26 呼吸波による動脈圧の変動 (Respiratory Waves in the Arterial Pressure)
- 27 動脈圧血管運動波—圧力反射制御システムの振動 (Arterial Pressure Vasomotor Waves—Oscillation of Pressure Reflex Control Systems)
循環の神経制御 (Nervous Regulation of the Circulation)
第17章で説明したように、体内の組織や臓器の血流の調整は主に局所組織制御機構 (local tissue control mechanisms) によるものです。本章では、循環の神経制御が、身体の異なる領域への血流再分配 (redistributing blood flow)、心臓のポンピング活動の増減 (increasing or decreasing pumping activity)、および全身動脈圧の迅速な制御 (rapid control of systemic arterial pressure) などのより全体的な機能 (global functions) を持つ方法について説明します。
神経系は、主に自律神経系 (autonomic nervous system) を通じて循環を制御します。このシステムの全体的な機能は第61章で説明されており、第17章でも導入されています。本章では、追加の具体的な解剖学的 (anatomical) および機能的特徴 (functional characteristics) について考察します。
自律神経系 (Autonomic Nervous System)
循環の調整において最も重要な自律神経系 (autonomic nervous system) の部分は、交感神経系 (sympathetic nervous system) です。しかしながら、副交感神経系 (parasympathetic nervous system)(より消化器で重要) も心臓機能の調整に重要な役割を果たします。
交感神経系 (Sympathetic Nervous System)
図18-1は、循環の交感神経制御の解剖学を示しています。交感神経の血管運動神経線維 (vasomotor nerve fibers) は胸部の全ての脊髄神経および1~2本の腰部脊髄神経 (lumbar spinal nerves) を通じて脊髄から出ます。次に、これらは直ちに脊柱の両側にある交感神経鎖 (sympathetic chain) に入ります。その後、2つの経路で循環に到達します。(1) 主に内臓および心臓の血管に支配する特定の交感神経 (specific sympathetic nerves) を通じて、(2) 周辺部に配布される脊髄神経の末端部分に直ちに入ります。
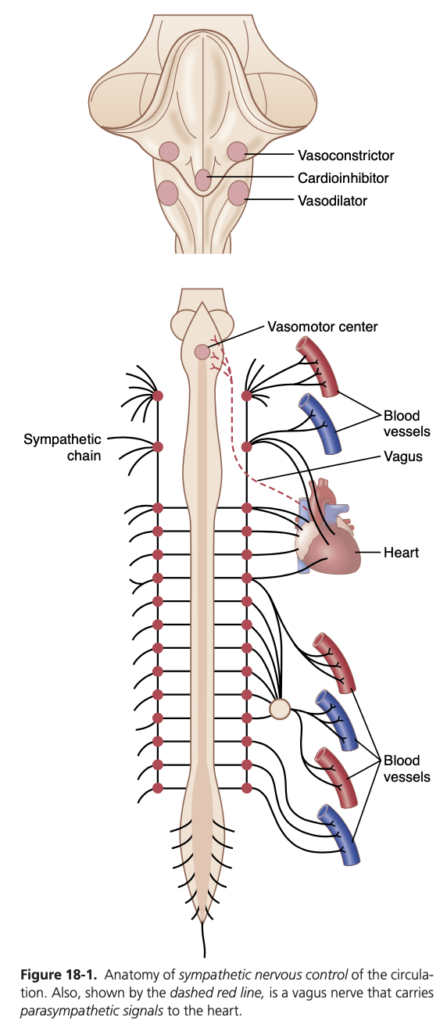
血管の交感神経支配 (Sympathetic Innervation of the Blood Vessels)
図18-2は、血管への交感神経線維の分布 (distribution of sympathetic nerve fibers) を示しており、ほとんどの組織において毛細血管 (capillaries) を除くすべての血管が支配されている (innervated) ことを示しています。
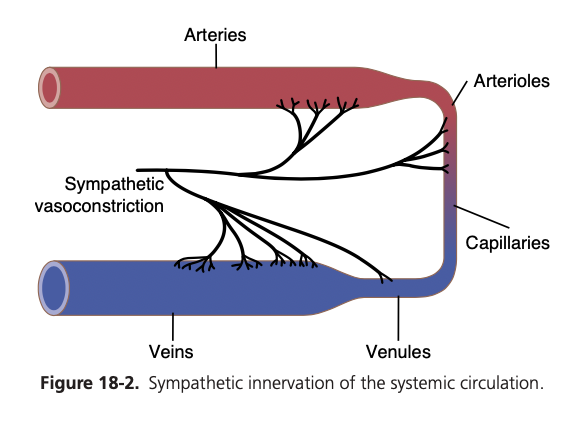
小動脈および細動脈への交感神経支配 (innervation) により、交感神経の刺激は血流抵抗 (resistance to blood flow) を増加させ、組織を通る血流速度を減少させることが可能です。また、大静脈などの大血管 (large vessels) への交感神経支配により、交感神経の刺激がこれらの血管の容量 (volume) を減少させ、心臓に血液を送り込むことが可能です。
交感神経刺激による心拍数と収縮力の増加 (Sympathetic Stimulation Increases Heart Rate and Contractility)
交感神経線維は心臓に直接到達し、図18-1に示されているように、交感神経刺激は心臓の活動 (activity) を著しく増加させ、心拍数を増加させると共にポンピング力 (pumping strength) を強化します。
副交感神経刺激による心拍数と収縮力の減少 (Parasympathetic Stimulation Decreases Heart Rate and Contractility)
副交感神経系は、消化器系の制御 (control of gastrointestinal actions) など多くの自律機能において極めて重要であるものの、大部分の組織における血管機能の調整 (regulation of vascular function) には重要な役割を果たしません。その最も重要な循環への影響は、迷走神経 (vagus nerves) を介して心拍数を制御することです。
交感神経血管収縮系と中枢神経系によるその制御 (Sympathetic Vasoconstrictor System and Its Control by the Central Nervous System)
交感神経は多数の血管収縮神経線維 (vasoconstrictor nerve fibers) と少数の血管拡張神経線維 (vasodilator fibers) を運びます。これらの血管収縮線維はほぼすべての循環系の領域に分布していますが、腎臓 (kidneys)、腸 (intestines)、脾臓 (spleen)、および皮膚 (skin) で特に強力です。一方で、骨格筋 (skeletal muscle)、心臓 (heart)、および脳 (brain) ではその作用はあまり強くありません。
脳の血管運動中枢とその血管収縮系の制御 (Vasomotor Center in the Brain and Its Control of the Vasoconstrictor System)
血管運動中枢 (vasomotor center) は主に延髄の網様体および橋の下三分の一に位置しています。この中枢は、迷走神経を通じて心臓に副交感神経インパルス (parasympathetic impulses) を送信し、また脊髄および末梢交感神経を通じてほぼ全身の動脈、細動脈、および静脈に交感神経インパルス (sympathetic impulses) を送信します。
血管運動中枢の全体的な組織構造はまだ明確ではありませんが、実験によりこの中枢におけるいくつかの重要な領域が特定されています。
- 血管収縮領域 (vasoconstrictor area) – 上部延髄の前外側部分に位置し、ここから出るニューロンは脊髄のすべてのレベルに分布し、交感神経系の節前収縮ニューロン (preganglionic vasoconstrictor neurons) を興奮させます。
- 血管拡張領域 (vasodilator area) – 下部延髄の前外側部分に位置し、ここから出る線維が血管収縮領域を抑制し、血管拡張 (vasodilation) を引き起こします。
- 感覚領域 (sensory area) – 延髄および橋の後外側部分に位置し、この領域のニューロンは主に迷走神経および舌咽神経を介して循環系からの感覚神経信号 (sensory nerve signals) を受け取り、これらの信号が血管収縮および血管拡張領域の活動を制御します。これにより、循環機能の反射制御が提供されます。
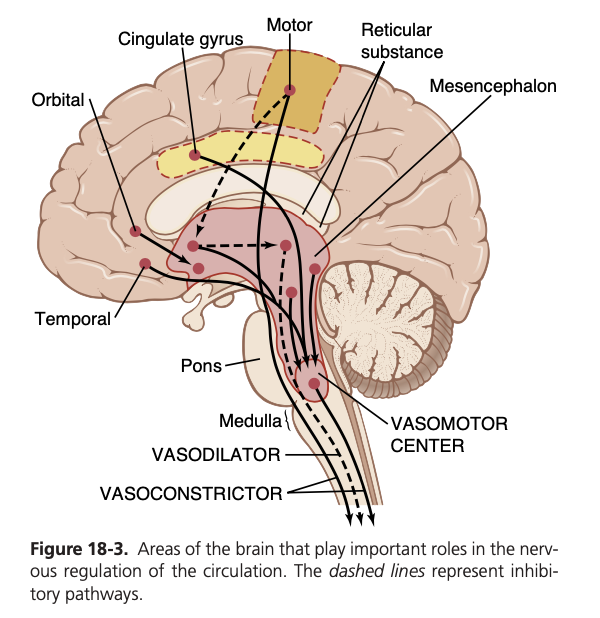
血管の持続的な部分的収縮—交感神経血管収縮トーン (Continuous Partial Constriction of Blood Vessels by Sympathetic Vasoconstrictor Tone)
通常の状態では、血管運動中枢の血管収縮領域は、全身の交感神経血管収縮神経線維 (sympathetic vasoconstrictor nerve fibers) に対して継続的に信号を送信し、これらの線維が約0.5~2回/秒の速度でゆっくりと発火します。この持続的な発火は交感神経血管収縮トーン (sympathetic vasoconstrictor tone) と呼ばれています。これらのインパルスは通常、血管の部分的な収縮状態 (partial state of constriction)、すなわち血管運動トーン (vasomotor tone) を維持します。
図18-4は、この血管収縮トーンの重要性を示しています。この図の実験では、動物に脊髄麻酔 (spinal anesthetic) が投与されました。この麻酔は脊髄から末梢への交感神経インパルスのすべての伝達を遮断しました。その結果、動脈圧 (arterial pressure) は100 mmHgから50 mmHgに低下し、全身の血管収縮トーンが失われた結果が示されています。数分後、少量のノルエピネフリン (norepinephrine) が血液中に注入されました(ノルエピネフリンは交感神経血管収縮神経線維の末端で分泌される主要な血管収縮ホルモン物質です)。この注入されたホルモンが血管に運ばれると、再び血管が収縮し、動脈圧は通常よりも高いレベルまで1~3分間上昇し、その後ノルエピネフリンが分解されました。
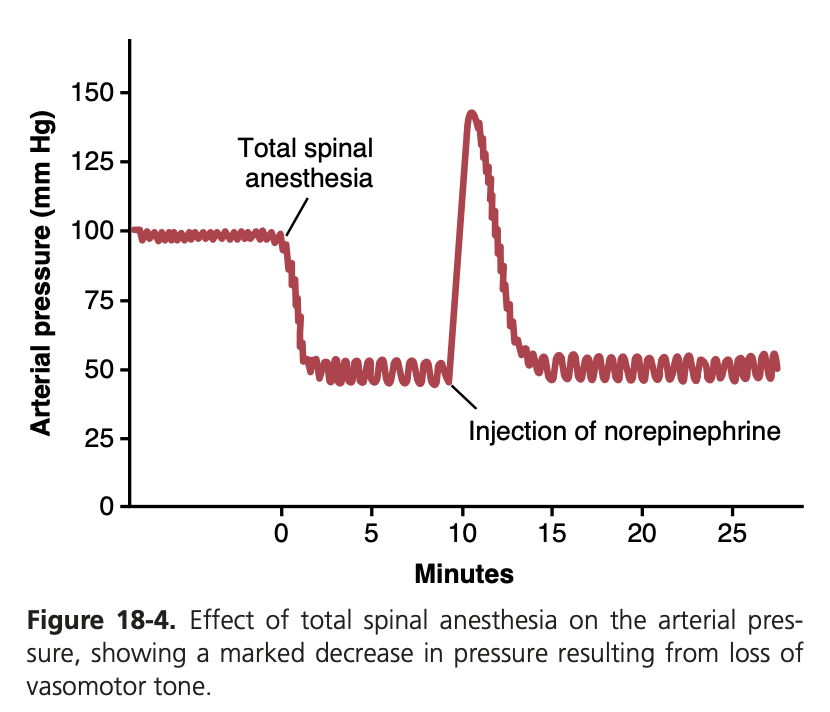
血管運動中枢による心臓活動の制御 (Control of Heart Activity by the Vasomotor Center)
血管運動中枢が血管収縮の度合いを調整するのと同時に、心臓の活動も制御しています。血管運動中枢の外側部分 (lateral portions) は、心拍数と収縮力を増加させる必要があるとき、交感神経線維を通じて心臓に興奮性インパルス (excitatory impulses) を送信します。逆に、心臓のポンピングを減少させる必要があるときには、血管運動中枢の内側部分 (medial portion) が迷走神経の背側運動核 (dorsal motor nuclei of the vagus nerves) に信号を送り、迷走神経を通じて心臓に副交感神経インパルス (parasympathetic impulses) を送信し、心拍数および収縮力を減少させます。したがって、血管運動中枢は心臓の活動を増加または減少させることができます。
高位神経中枢による血管運動中枢の制御 (Control of the Vasomotor Center by Higher Nervous Centers)
橋 (pons)、中脳 (mesencephalon)、間脳 (diencephalon) の網様体 (reticular substance) に存在する多数の小さなニューロンが、血管運動中枢を興奮 (excite) または抑制 (inhibit) することができます。この網様体は図18-3に示されています。一般的に、網様体の外側および上部 (lateral and superior portions) にあるニューロンは興奮 (excitation) を引き起こし、内側および下部 (medial and inferior portions) にあるニューロンは抑制 (inhibition) を引き起こします。
視床下部 (hypothalamus) は血管収縮系の制御において特別な役割を果たし、血管運動中枢に対して強力な興奮性 (excitatory) または抑制性 (inhibitory) の影響を及ぼすことができます。視床下部の後外側部分 (posterolateral portions) は主に興奮を引き起こし、前部 (anterior portion) は刺激された正確な部分に応じて軽い興奮または抑制を引き起こすことができます。
また、大脳皮質 (cerebral cortex) の多くの部分も血管運動中枢を興奮または抑制することができます。例えば、運動皮質 (motor cortex) の刺激は、視床下部を通じて血管運動中枢に伝達されるインパルスにより血管運動中枢を興奮させます。また、前頭葉眼窩部 (orbital areas of the frontal cortex)、前帯状回 (anterior part of the cingulate gyrus)、扁桃体 (amygdala)、中隔 (septum)、および海馬 (hippocampus) の刺激も、これらの領域の正確な部分や刺激の強度に応じて血管運動中枢を興奮または抑制します。したがって、脳の広範な基底部が心血管機能 (cardiovascular function) に重大な影響を及ぼす可能性があります。
交感神経血管収縮神経伝達物質—ノルエピネフリン (Norepinephrine Is the Sympathetic Vasoconstrictor Neurotransmitter)
血管収縮神経 (vasoconstrictor nerves) の末端で分泌される物質は、ほとんどがノルエピネフリン (norepinephrine) であり、これは血管平滑筋のアルファアドレナリン受容体 (alpha-adrenergic receptors) に直接作用して血管収縮 (vasoconstriction) を引き起こします。
副腎髄質と交感神経血管収縮系との関係 (Adrenal Medullae and Their Relationship to the Sympathetic Vasoconstrictor System)
交感神経インパルス (sympathetic impulses) は、血管に送られるのと同時に副腎髄質 (adrenal medullae) にも伝達されます。これらのインパルスは、副腎髄質からエピネフリン (epinephrine) とノルエピネフリン (norepinephrine) を循環血中に分泌させます。この2つのホルモンは血流によって全身に運ばれ、通常、すべての血管に直接作用して血管収縮 (vasoconstriction) を引き起こします。しかし、一部の組織ではエピネフリン (epinephrine) がベータアドレナリン受容体 (beta-adrenergic receptors) を刺激し、これにより血管が収縮するのではなく血管拡張 (vasodilation) します。
交感神経血管拡張系とその中枢神経系による制御 (Sympathetic Vasodilator System and Its Control by the Central Nervous System)
骨格筋 (skeletal muscles) への交感神経は、交感神経血管拡張線維 (sympathetic vasodilator fibers) と収縮線維 (constrictor fibers) の両方を運びます。猫などの一部の動物では、これらの拡張線維は末端でアセチルコリン (acetylcholine) を放出しますが、霊長類では血管拡張効果は筋肉の血管におけるベータアドレナリン受容体 (beta-adrenergic receptors) を刺激するエピネフリン (epinephrine) によるものと考えられています。
図18-3の破線で示されているように、この血管拡張系の中枢神経系による制御経路が存在します。この系を制御する脳の主要な領域は視床下部の前部 (anterior hypothalamus) です。
交感神経血管拡張系の役割 (Possible Role of the Sympathetic Vasodilator System)
交感神経血管拡張系 (sympathetic vasodilator system) は、人間の循環制御には大きな役割を果たしていないようです。筋肉への交感神経が完全に遮断されても、多くの生理的状態において筋肉自身が血流を制御する能力にはほとんど影響を及ぼしません。しかし、一部の実験では、運動の開始時に交感神経系が筋肉の初期血管拡張 (vasodilation) を引き起こし、筋肉が栄養を必要とする前に血流を予測的に増加させる可能性があることを示唆しています。この交感神経血管拡張反応 (sympathetic vasodilator response) は、エピネフリン (epinephrine) がベータアドレナリン受容体 (beta-adrenergic receptors) を刺激すること、またはアセチルコリン (acetylcholine) による一酸化窒素 (nitric oxide) の血管内皮からの放出によって媒介される可能性があります。
感情的失神—血管迷走神経性失神 (Emotional Fainting—Vasovagal Syncope)
強い感情的動揺 (emotional disturbances) を経験した際に発生する興味深い血管拡張反応 (vasodilatory reaction) があります。この場合、筋肉血管拡張系 (muscle vasodilator system) が活性化され、同時に迷走神経心抑制中枢 (vagal cardioinhibitory center) が心臓に強い信号を送り、心拍数を著しく減少させます。その結果、動脈圧 (arterial pressure) は急速に低下し、脳への血流が減少し、意識を失います。この全体的な効果は血管迷走神経性失神 (vasovagal syncope) と呼ばれています。感情的失神は、大脳皮質での不安定な思考から始まります。経路はおそらく前部視床下部の血管拡張中枢を通り、次に延髄の迷走中枢を通り、迷走神経を通じて心臓に到達し、さらに脊髄を経由して筋肉の交感神経血管拡張神経に伝達されます。
動脈圧の迅速な制御における神経系の役割 (Role of the Nervous System in Rapid Control of Arterial Pressure)
循環の神経制御 (nervous control of the circulation) の最も重要な機能の1つは、動脈圧を迅速に上昇させる能力です。この目的のために、交感神経系の血管収縮 (vasoconstrictor) および心拍加速 (cardioaccelerator) の機能が一緒に刺激されます。同時に、心臓に対する副交感神経迷走神経の抑制信号 (inhibitory signals) が抑制されます。したがって、以下の3つの主要な変化が同時に発生し、それぞれが動脈圧の上昇に寄与します。
- 全身循環のほとんどの細動脈 (arterioles of the systemic circulation) が収縮し、これにより総末梢抵抗 (total peripheral resistance) が大幅に増加し、動脈圧が上昇します。
- 特に静脈 (veins) が強く収縮します。この収縮は、大きな末梢血管 (large peripheral blood vessels) から心臓に血液を押し出し、心臓室内の血液量を増加させます。この心臓の伸展により、心臓はより強く拍動し、より多くの血液をポンピングすることができます。このことも動脈圧の上昇に寄与します。
- 最後に、心臓は自律神経系によって直接刺激され、心臓ポンピング (cardiac pumping) がさらに強化されます。この強化の多くは心拍数の増加 (increase in heart rate) によるもので、場合によっては通常の3倍に増加します。また、交感神経信号が直接心筋の収縮力を増加させ、心臓がより多くの血液をポンピングする能力を高めます。強い交感神経刺激があるとき、心臓は通常の約2倍の量の血液をポンピングすることができ、急性の動脈圧上昇にさらに寄与します。
動脈圧の神経制御の迅速さ (Nervous Control of Arterial Pressure Is Rapid)
動脈圧の神経制御 (nervous control of arterial pressure) の特に重要な特性は、その反応の迅速性 (rapidity of response) です。この反応は数秒以内に始まり、圧力を5〜10秒で通常の2倍にまで増加させることがあります。逆に、神経性心血管刺激の急な抑制は、動脈圧を10〜40秒で半分以下に低下させることができます。したがって、神経制御は動脈圧調整の最も迅速なメカニズムです。
筋肉運動および他のストレス時の動脈圧の上昇 (Increases in Arterial Pressure During Muscle Exercise and Other Stresses)
神経系 (nervous system) の動脈圧上昇能力の重要な例は、筋肉運動 (muscle exercise) 中に発生する圧力の上昇です。重い運動中、筋肉は大幅に増加した血流 (blood flow) を必要とします。この増加の一部は、筋肉細胞の代謝の増加によって引き起こされる筋肉血管の局所血管拡張 (local vasodilation) によります。また、運動中に全体の循環 (overall circulation) の交感神経刺激 (sympathetic stimulation) によって引き起こされる動脈圧の同時上昇 (simultaneous elevation of arterial pressure) も寄与します。激しい運動中には動脈圧が約30〜40%上昇し、それによって血流がほぼ2倍に増加します。
多くの他のストレスの種類においても同様の圧力上昇が発生することがあります。例えば、極度の恐怖に陥った場合、動脈圧は数秒以内に75〜100 mmHg上昇することがあります。この反応は警報反応 (alarm reaction) と呼ばれ、危険から逃れるために必要な筋肉に即座に血液を供給できるようにするための圧力上昇を提供します。
動脈圧を正常に保つための反射機構 (Reflex Mechanisms for Maintaining Normal Arterial Pressure)
自律神経系の動脈圧を上昇させる機能 (functions to increase arterial pressure) とは別に、動脈圧を正常に近い状態に保つために常時作動している複数の無意識の特殊な神経制御機構 (subconscious special nervous control mechanisms) があります。これらはほぼすべて負のフィードバック反射機構 (negative feedback reflex mechanisms) であり、以下のセクションで説明されています。
バロレセプター動脈圧制御システム—バロレセプター反射 (Baroreceptor Arterial Pressure Control System—Baroreceptor Reflexes) バロ:圧
動脈圧制御の神経メカニズム (nervous mechanisms) の中で最もよく知られているものはバロレセプター反射 (baroreceptor reflex) です。基本的に、この反射は大きな全身動脈の壁の特定の地点に位置する伸展受容体 (stretch receptors)、すなわちバロレセプター (baroreceptors) によって引き起こされます。動脈圧の上昇はバロレセプターを伸展させ、それにより中枢神経系 (CNS) に信号を送信します。その後、フィードバック信号が自律神経系を通じて循環に戻り、動脈圧を正常レベルに向けて減少させます。
バロレセプターの生理学的解剖学とその神経支配 (Physiologic Anatomy of the Baroreceptors and Their Innervation)
バロレセプター (baroreceptors) は、動脈の壁にあるスプレー型神経終末 (spray-type nerve endings) であり、伸展されることで刺激されます。バロレセプターの一部は胸部および頸部のほぼすべての大動脈の壁に存在していますが、図18-5に示されているように、特に以下の領域に多く存在しています。
- 頸動脈洞 (carotid sinus) – 各内頸動脈 (internal carotid artery) の壁、頸動脈分岐の少し上に位置する領域。
- 大動脈弓 (aortic arch) の壁。
図18-5に示されているように、頸動脈バロレセプターからの信号は小さなヘーリング神経 (Hering’s nerves) を介して舌咽神経 (glossopharyngeal nerves) に伝達され、その後延髄 (medulla) の孤束核 (nucleus tractus solitarius) に到達します。また、大動脈バロレセプターからの信号は、迷走神経 (vagus nerves) を介して同じ孤束核に伝達されます。
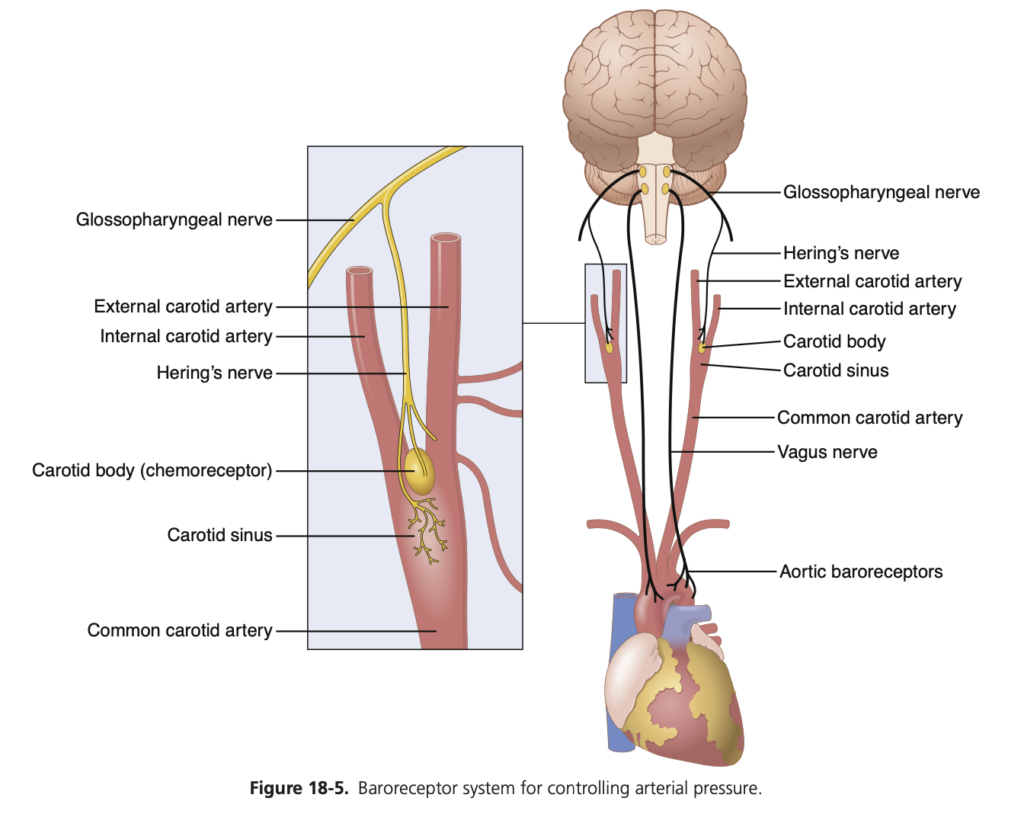
動脈圧の変化に対するバロレセプターの応答 (Response of the Baroreceptors to Changes in Arterial Pressure)
図18-6は、異なる動脈圧レベルがヘーリングの頸動脈洞神経 (Hering’s carotid sinus nerve) のインパルス伝達速度に与える影響を示しています。頸動脈洞バロレセプターは0〜50〜60 mmHgの圧力ではまったく刺激されませんが、それ以上のレベルでは徐々に応答し、約180 mmHgで最大に達します。大動脈バロレセプターの応答も頸動脈受容体の応答と類似していますが、一般的に動脈圧レベルは約30 mmHg高いところで動作します。
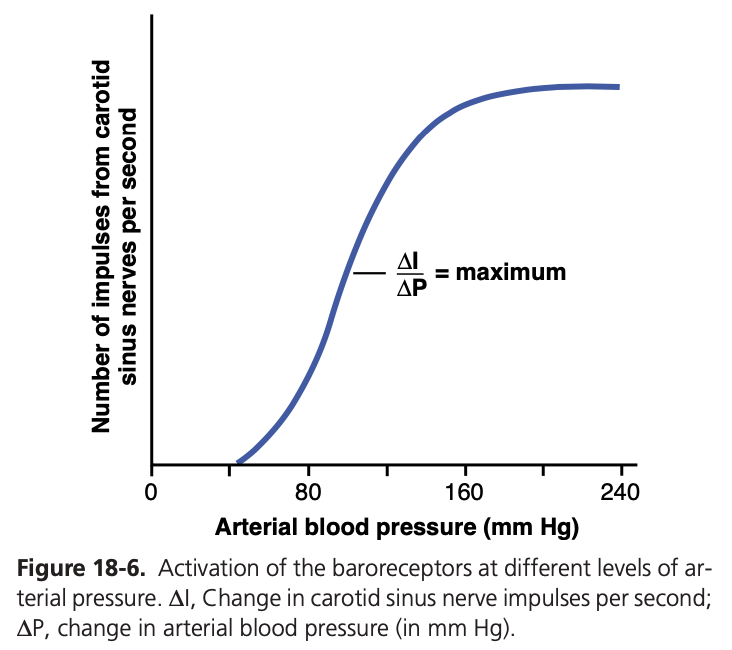
バロレセプターによって引き起こされる循環反射 (Circulatory Reflex Initiated by the Baroreceptors)
バロレセプター信号が延髄の孤束核 (nucleus tractus solitarius of the medulla) に入った後、二次信号は延髄の血管収縮中枢 (vasoconstrictor center) を抑制し、迷走副交感神経中枢 (vagal parasympathetic center) を興奮させます。その結果、以下のような効果があります。
- 末梢循環系 (peripheral circulatory system) 全体の静脈および細動脈の拡張 (vasodilation of the veins and arterioles)。
- 心拍数 (heart rate) の減少および心臓の収縮力 (strength of heart contraction) の減少。
したがって、バロレセプターが動脈の高圧によって興奮すると、反射的に動脈圧 (arterial pressure) が低下し、これには末梢抵抗 (peripheral resistance) の低下と心拍出量 (cardiac output) の減少が関与しています。逆に、低圧では反対の効果があり、反射的に圧力が正常に戻る方向に上昇します。
図18-7は、両側の総頸動脈 (common carotid arteries) を閉塞することによって引き起こされた動脈圧の典型的な反射的変化を示しています。この操作により頸動脈洞圧 (carotid sinus pressure) が減少し、バロレセプターからの信号が減少して血管運動中枢への抑制効果が弱くなります。その結果、血管運動中枢が通常よりも活発になり、閉塞されている10分間にわたり大動脈圧 (aortic arterial pressure) が上昇し続けます。閉塞を解除すると、頸動脈洞の圧力が上昇し、頸動脈洞反射によって大動脈圧はほぼ直ちに正常よりもわずかに低下し、一時的な過補償の後、再び正常に戻ります。
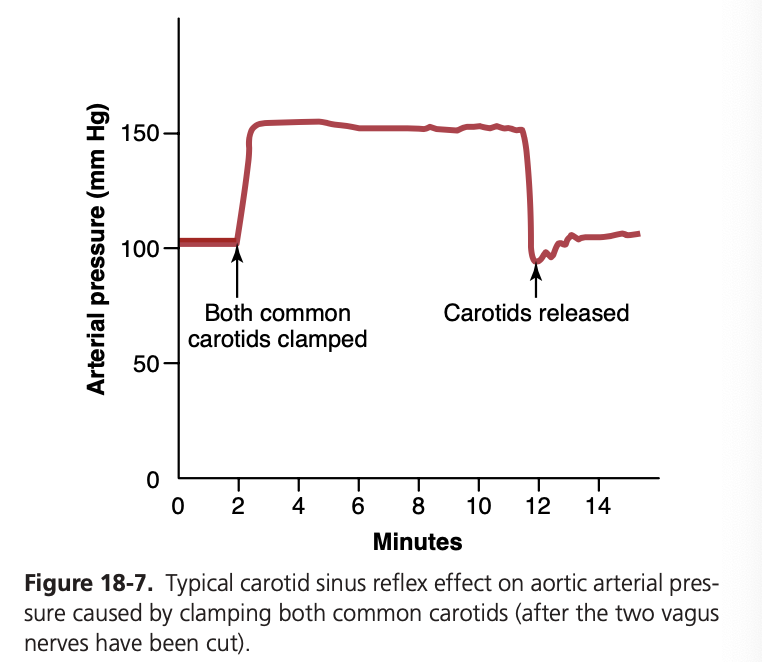
体位変化時の血圧変動を緩和するバロレセプター (Baroreceptors Attenuate Blood Pressure Changes During Changes in Body Posture)
バロレセプター (baroreceptors) が体の上部の動脈圧を比較的一定に保つ能力は、立ち上がったとき (standing up) に重要です。横たわった状態から立ち上がると、頭部および体上部の動脈圧が低下する傾向があり、この圧力の著しい減少は意識喪失 (loss of consciousness) を引き起こす可能性があります。しかし、バロレセプターでの圧力低下は即座に反射を誘発し、体全体で強い交感神経放出を引き起こし、頭部および体上部の圧力の低下を最小限に抑えます。
バロレセプター制御システムの圧力緩衝機能 (Pressure Buffer Function of the Baroreceptor Control System)
バロレセプターシステムは動脈圧の上昇や低下に抵抗する (opposes increases or decreases in arterial pressure) ため、圧力緩衝システム (pressure buffer system) と呼ばれています。また、バロレセプターからの神経は緩衝神経 (buffer nerves) と呼ばれます。
図18-8は、この緩衝機能の重要性を示しています。上のパネルは正常な犬の2時間の動脈圧記録を示しており、下のパネルは頸動脈洞 (carotid sinuses) および大動脈 (aorta) からのバロレセプター神経が除去された犬の動脈圧記録を示しています。バロレセプターが正常に機能している場合、動脈圧は85〜115 mmHgの狭い範囲に保たれ、ほとんどの場合約100 mmHgです。しかし、バロレセプターが除去された場合、頻度分布曲線が平坦になり、圧力範囲が2.5倍に広がり、しばしば50 mmHgにまで低下したり、160 mmHgを超えることもあります。したがって、バロレセプターシステムが存在しない場合、圧力の変動が極めて大きくなることがわかります。バロレセプターシステムの主な目的は、動脈圧の瞬間的な変動を3分の1に減少させることです。
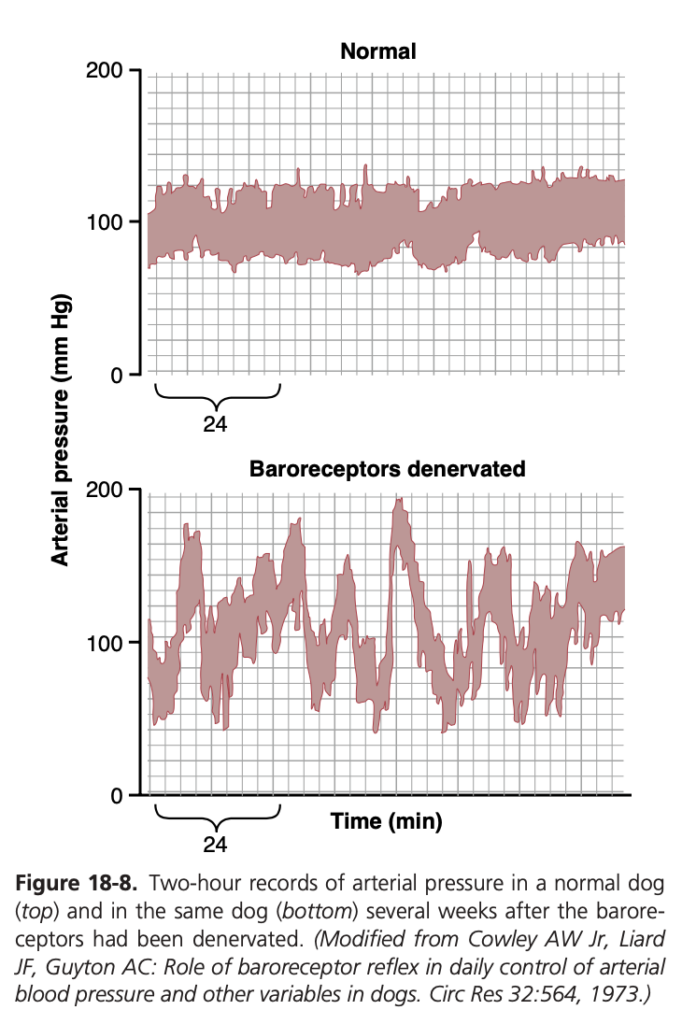

長期的な動脈圧調整におけるバロレセプターの重要性 (Are the Baroreceptors Important in Long-Term Regulation of Arterial Pressure?)
動脈バロレセプター (arterial baroreceptors) は強力な瞬間的な動脈圧制御を提供しますが、長期的な血圧調整におけるその重要性については議論があります。バロレセプターが長期間の動脈圧調整に比較的重要でないと考えられている理由の1つは、それらが1〜2日以内にそれらが露出した圧力レベルにリセットされる傾向 (resetting tendency) があるからです。すなわち、動脈圧が通常の100 mmHgから160 mmHgに上昇すると、最初は非常に高い頻度でバロレセプターからインパルスが送信されます。しかし、その後数分で発火率は大幅に減少し、さらに次の1〜2日間で発火率はかなりゆっくりと減少し、最終的には圧力が160 mmHgであってもほぼ正常に戻ります。逆に、動脈圧が非常に低いレベルに低下すると、バロレセプターは最初はインパルスをまったく送信しませんが、1〜2日間で発火率が制御レベルに向かって徐々に回復します。
このリセット (resetting) により、バロレセプターは長期間の圧力変化を修正するための制御システムとしての有効性が低下する可能性があります。しかし、実験的研究では、バロレセプターは完全にリセットされるわけではなく、特に腎臓の交感神経活動に影響を与えることによって長期的な血圧調整に寄与する可能性が示唆されています。例えば、動脈圧が長期間上昇すると、バロレセプター反射は腎臓の交感神経活動の減少を媒介し、腎臓によるナトリウムおよび水の排泄 (excretion of sodium and water) を促進します。この作用により、血液量 (blood volume) が徐々に減少し、動脈圧が正常に戻るのを助けます。したがって、バロレセプターによる長期的な平均動脈圧 (mean arterial pressure) の調整には、主に腎体液圧力制御システム(およびその関連する神経およびホルモンメカニズム)との相互作用が必要です。
実験的研究および臨床試験では、頸動脈洞 (carotid sinus) の求心性神経線維の慢性的な電気刺激が交感神経活動および動脈圧を15〜20 mmHg以上持続的に低下させることが示されています。これらの観察結果は、持続的な動脈圧の上昇、すなわち慢性高血圧において生じるバロレセプター反射のリセットのほとんど、あるいはすべてが、中央神経系の血管運動中枢のリセットではなく、頸動脈洞神経の機械受容体 (carotid sinus nerve mechanoreceptors) そのもののリセットによることを示唆しています。
頸動脈および大動脈の化学受容体による動脈圧の制御—低酸素の動脈圧への影響 (Control of Arterial Pressure by the Carotid and Aortic Chemoreceptors—Effect of Low Oxygen on Arterial Pressure)
バロレセプターの圧力制御システムと密接に関連しているのは、バロレセプター反射とほぼ同様に機能する化学受容体反射 (chemoreceptor reflex) です。ただし、化学受容体 (chemoreceptors) は、伸展受容体の代わりにこの応答を開始します。
化学受容体細胞 (chemoreceptor cells) は、低酸素 (low oxygen)、高二酸化炭素 (elevated carbon dioxide)、および水素イオンの増加 (elevated hydrogen ion levels) に敏感です。これらは2ミリメートルほどの小さな化学受容体器官に位置し、それぞれが頸動脈体 (carotid bodies) と大動脈体 (aortic bodies) として知られています。頸動脈体は各総頸動脈の分岐部 (bifurcation of each common carotid artery) に1つずつ、通常1〜3個の大動脈体が大動脈の近くに存在します。※圧はcarotid sinus!! bodiesではない!
化学受容体からの信号は、バロレセプター線維と共にヘーリング神経 (Hering’s nerves) および迷走神経 (vagus nerves) を通じて延髄 (vasomotor center of the brain stem) に送られます。
化学受容器による動脈圧制御のメカニズム (Mechanism of Arterial Pressure Control by Chemoreceptors)
各頸動脈体 (carotid body) または大動脈体 (aortic body) は、小さな栄養動脈を通じて豊富な血流 (blood flow) を受けています。そのため、化学受容器は常に動脈血 (arterial blood) と密接に接触しています。動脈圧が臨界レベル (critical level) 以下に低下すると、化学受容器が刺激されます。これは、血流の減少により酸素の供給が減少し、二酸化炭素 (carbon dioxide) と水素イオン (hydrogen ions) が蓄積するためです。
化学受容器から伝達される信号は血管運動中枢 (vasomotor center) を興奮させ、この応答により動脈圧を正常に戻す方向に上昇させます。ただし、この化学受容器反射 (chemoreceptor reflex) は、動脈圧が80 mmHgを下回るまでは強力な動脈圧制御器ではありません。そのため、この反射は圧力が低い状態でさらなる動脈圧の低下を防ぐために重要となります。
化学受容器については、第42章で呼吸制御 (respiratory control) に関連してより詳細に説明されています。通常、化学受容器は血圧制御よりも呼吸制御においてはるかに重要な役割を果たします。しかし、化学受容器の活性化は、重度の肥満 (severe obesity) や閉塞性睡眠時無呼吸 (obstructive sleep apnea) のような状態でも動脈圧の上昇に寄与することがあります。閉塞性睡眠時無呼吸は、夜間の呼吸停止と低酸素症が繰り返される重篤な睡眠障害です。
心房および肺動脈反射による動脈圧の調整 (Atrial and Pulmonary Artery Reflexes Regulate Arterial Pressure)
心房 (atria) および肺動脈 (pulmonary arteries) には、低圧受容器 (low-pressure receptors) と呼ばれる伸展受容器 (stretch receptors) が壁に存在します。これらは大動脈のバロレセプター (baroreceptor stretch receptors) と似ています。これらの低圧受容器 (low-pressure receptors) は、血液量の変化に対する動脈圧の変化を最小限に抑えるうえで重要な役割を果たします。
例えば、犬に300ミリリットルの血液を急速に注入すると、受容器がすべて正常であれば動脈圧 (arterial pressure) は約15 mmHgしか上昇しません。しかし、動脈バロレセプターが除去されると、圧力は約40 mmHg上昇します。さらに、低圧受容器も除去されると、動脈圧は約100 mmHgまで上昇します。
したがって、低圧受容器は全身動脈圧を検出できないにもかかわらず、血液量の増加 (increase in blood volume) によって同時に引き起こされる低圧領域 (low-pressure areas) の圧力増加を検出し、バロレセプター反射と並行した反射を誘発して動脈圧の制御力を強化します。
心房反射による腎臓の活性化—容積反射 (Atrial Reflexes That Activate the Kidneys—The Volume Reflex)
心房の伸展 (stretch of the atria) および低圧心房受容器 (low-pressure atrial receptors) の活性化は、腎交感神経活動 (renal sympathetic nerve activity) の反射的な減少、尿細管再吸収 (tubular reabsorption) の減少、および腎臓における輸入細動脈の拡張 (dilation of afferent arterioles) を引き起こします(図18-10)。また、心房から視床下部に信号が送られ、抗利尿ホルモン (antidiuretic hormone, ADH) の分泌を減少(尿量を増加させる)させます。腎臓の輸入細動脈の抵抗低下 (decreased afferent arteriolar resistance) により糸球体毛細血管圧 (glomerular capillary pressure) が上昇し、腎細管への濾過量 (filtration of fluid) が増加します。また、ADHレベルの減少により、腎細管からの水の再吸収 (reabsorption of water) が減少します。これらの作用の組み合わせにより、腎臓からの体液の排出 (fluid loss) が増加し、血液量の増加 (increased blood volume) が緩和されます。心房伸展 (atrial stretch) によっても心房性ナトリウム利尿ペプチド (atrial natriuretic peptide) の放出が引き起こされ、さらにナトリウムおよび水の排泄 (excretion of sodium and water) を促進し、血液量を正常に戻す働きがあります(図18-10参照)。
これらのメカニズムは、血液量を元に戻すだけでなく、間接的に圧力制御 (pressure controllers) としても機能します。なぜなら、過剰な血液量 (excess volume) が心臓を強く拍動させ、心拍出量 (cardiac output) を増加させ、動脈圧を高めるからです。この容積反射機構 (volume reflex mechanism) については、第30章で他の血液量制御メカニズムと共に再度説明されています。
心房圧の増加による心拍数の増加—ベインブリッジ反射 (Increased Atrial Pressure Raises Heart Rate—Bainbridge Reflex)
心房圧 (atrial pressure) の増加により、特に既存の心拍数が遅い場合 (prevailing heart rate is slow)、心拍数が最大75%増加することがあります。心拍数が速い場合には、体液の注入によって引き起こされる心房伸展は、動脈バロレセプターの活性化により心拍数を減少させる可能性があります。したがって、心血量の増加および心房伸展が心拍数に与える影響は、バロレセプター反射 (baroreceptor reflexes)(心拍数を減少させる傾向)とベインブリッジ反射 (Bainbridge reflex)(心拍数を増加させる傾向)の相対的な寄与に依存します(図18-10参照)。血液量が正常を超えて増加すると、ベインブリッジ反射はバロレセプター反射 (baroreflexes) の抑制作用にもかかわらず心拍数を増加させることがよくあります。
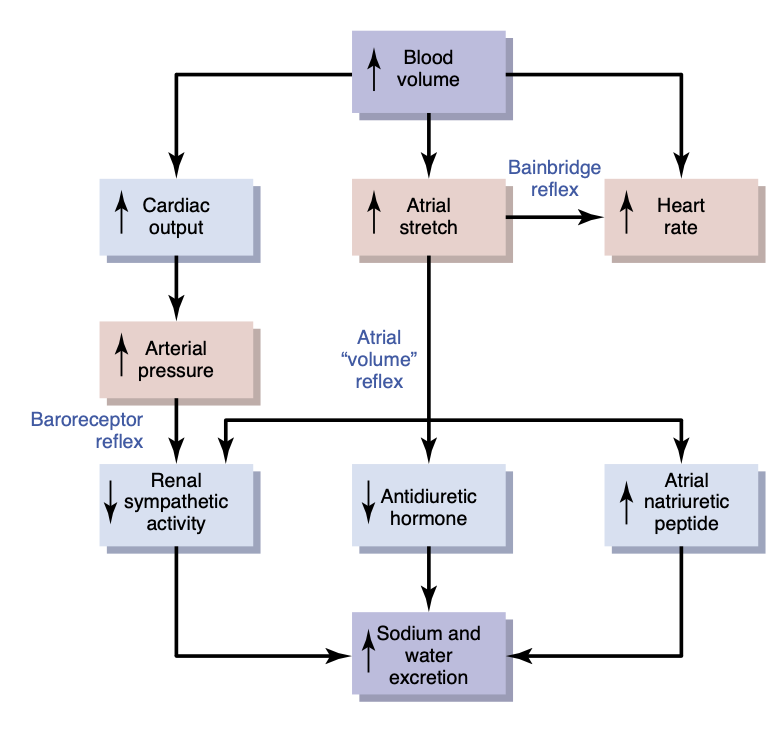
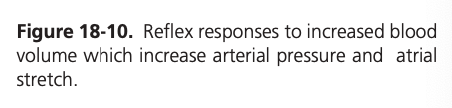
心房量の増加 (increased atrial volume) によって引き起こされる心拍数の一部は、洞房結節 (sinus node) の伸展による直接的な効果によるものであり、第10章で述べたように、こうした直接的な伸展により心拍数が最大15%増加する可能性があります。さらに、ベインブリッジ反射 (Bainbridge reflex) により心拍数が40〜60%増加します。この反射を誘発する心房の伸展受容器は、その求心性信号 (afferent signals) を迷走神経 (vagus nerves) を介して脳の延髄に送ります。その後、遠心性信号 (efferent signals) が迷走神経および交感神経を介して伝達され、心拍数および収縮力が増加します。この反射は、静脈、心房、および肺循環における血液のうっ滞を防ぐのに役立ちます。
脳血管運動中枢への血流減少による動脈圧の上昇—中枢神経系虚血反応 (Decreased Blood Flow to Brain Vasomotor Center Elicits Increased Blood Pressure—CNS Ischemic Response)
血圧の神経制御のほとんどは、バロレセプター (baroreceptors)、化学受容器 (chemoreceptors)、および低圧受容器 (low-pressure receptors) に由来する反射によって達成され、これらはすべて脳の外の末梢循環系 (peripheral circulation) に位置しています。しかし、脳幹下部 (lower brain stem) にある血管運動中枢 (vasomotor center) への血流が著しく減少し、栄養不足—すなわち脳虚血 (cerebral ischemia)—を引き起こすと、血管運動中枢の血管収縮および心拍加速ニューロン (vasoconstrictor and cardioaccelerator neurons) が直接反応し、強く興奮します。この興奮が発生すると、全身動脈圧 (systemic arterial pressure) は心臓が可能な限り高いレベルまで上昇することがあります。この効果は、流れが遅くなった血液 (slowly flowing blood) が二酸化炭素 (carbon dioxide) を脳幹血管運動中枢から運び去ることができなくなることが原因であると考えられています。血管運動中枢への血流が低レベルになると、局所的な二酸化炭素濃度が著しく増加し、脳の延髄 (medulla) にある交感神経血管運動制御領域を強力に刺激します。
他にも、乳酸 (lactic acid) やその他の酸性物質の蓄積が血管運動中枢を強力に刺激し、動脈圧を上昇させる可能性があります。この動脈圧の上昇は中枢神経系虚血反応 (CNS ischemfic response) として知られています。
中枢神経系虚血反応が動脈圧の調整者としての重要性 (Importance of CNS Ischemic Response as a Regulator of Arterial Pressure)
中枢神経系虚血反応の強力な性質にもかかわらず、この反応は動脈圧が60 mmHg以下に低下し、15〜20 mmHgに達したときに最も強力になります。そのため、中枢神経系虚血反応は通常の動脈圧調整のためのメカニズムの1つではありません。むしろ、血流が危険なレベルに近づくときに、さらなる動脈圧低下を防ぐための緊急圧力制御システムとして主に機能します。この反応は「最後の砦の圧力制御メカニズム (last-ditch stand pressure control mechanism)」とも呼ばれています。
脳周囲の圧力増加に対するクッシング反応 (Cushing Reaction to Increased Pressure Around the Brain)
クッシング反応 (Cushing reaction) は、脳の頭蓋内空間 (cranial vault) における脳脊髄液圧 (cerebrospinal fluid pressure) の増加に起因する中枢神経系虚血反応の特殊なタイプです。例えば、脳脊髄液圧が動脈圧と同等に上昇すると、脳全体および脳内の動脈が圧迫され、脳への血流が遮断されます。この作用により中枢神経系虚血反応が引き起こされ、動脈圧が上昇します。動脈圧が脳脊髄液圧を上回るレベルに達すると、脳の血管に再び血液が流れ込み、脳虚血を緩和します。通常、血圧は脳脊髄液圧をわずかに上回る新たな平衡レベルに達し、これにより脳への血流が再開されます。クッシング反応は、脳の重要な中枢が栄養を失わないようにするため、脳脊髄液圧が脳動脈を圧迫するほど高くなった場合に保護的な役割を果たします。
動脈圧の神経制御における特殊な特徴 (Special Features of Nervous Control of Arterial Pressure)
骨格神経および骨格筋が心拍出量および動脈圧を増加させる役割 (Role of the Skeletal Nerves and Skeletal Muscles in Increasing Cardiac Output and Arterial Pressure)
循環の急速な神経制御のほとんどは自律神経系 (autonomic nervous system) を通じて行われますが、骨格神経 (skeletal nerves) および骨格筋 (skeletal muscles) も循環反応において主要な役割を果たす状況が少なくとも2つ存在します。
腹部圧縮反射による心拍出量および動脈圧の増加 (Abdominal Compression Reflex Increases Cardiac Output and Arterial Pressure)
バロレセプター (baroreceptor) または化学受容器 (chemoreceptor) の反射が引き起こされると、神経信号が骨格神経 (skeletal nerves) を通じて身体の骨格筋、特に腹筋 (abdominal muscles) に伝達されます。筋収縮 (muscle contraction) によって腹部の静脈貯蔵部 (venous reservoirs) が圧迫され、これにより腹部血管貯蔵部から心臓に向かって血液が移動します。その結果、心臓がポンピングできる血液量が増加します。この全体的な反応は腹部圧縮反射 (abdominal compression reflex) と呼ばれます。この反射の循環に対する効果は、静脈を収縮させる交感神経血管収縮インパルスによって引き起こされる効果と同じであり、心拍出量 (cardiac output) および動脈圧 (arterial pressure) の増加をもたらします。腹部圧縮反射は、過去に考えられていたよりもはるかに重要である可能性があります。骨格筋が麻痺した人々が正常な骨格筋を持つ人々に比べて低血圧エピソードを起こしやすいことがよく知られているためです。
運動中の骨格筋収縮が心拍出量および動脈圧を増加させる (Skeletal Muscle Contraction Increases Cardiac Output and Arterial Pressure During Exercise)
運動中に骨格筋 (skeletal muscles) が収縮すると、全身の血管が圧迫されます。運動を見越して筋肉を引き締めるだけでも、筋肉および腹部の血管が圧迫されます。この圧迫により、末梢血管から心臓および肺へ血液が移動し、心拍出量が増加します。この効果は、重い運動中に発生する心拍出量の5倍から7倍の増加 (fivefold to sevenfold increase in cardiac output) を引き起こすうえで不可欠です。心拍出量の増加は、運動中に動脈圧 (arterial pressure) を正常な100 mmHgから130〜160 mmHgに増加させるための重要な要素です。
呼吸波による動脈圧の変動 (Respiratory Waves in the Arterial Pressure)
各呼吸サイクル (respiratory cycle) に伴い、動脈圧は通常4〜6 mmHgの波のように上昇および下降します。これにより呼吸波 (respiratory waves) が動脈圧に生じます。この波は、いくつかの異なる効果によって生じ、そのうちの一部は反射的 (reflex in nature) なものです。
- 多くの呼吸シグナル (breathing signals) は、延髄の呼吸中枢 (respiratory center) から発生し、各呼吸サイクルと共に血管運動中枢 (vasomotor center) にも影響を与えます。
- 吸気を行うたびに、胸腔内の圧力が通常よりも負の方向に変化し、胸部内の血管が拡張します。これにより、左心房に戻る血液の量が減少し、一時的に心拍出量 (cardiac output) および動脈圧 (arterial pressure) が低下します。
- 呼吸による胸部血管の圧力変化が、血管および心房の伸展受容器 (vascular and atrial stretch receptors) を刺激することがあります。
動脈圧血管運動波—圧力反射制御システムの振動 (Arterial Pressure Vasomotor Waves—Oscillation of Pressure Reflex Control Systems)
動脈圧を記録する際、呼吸による小さな圧力波 (small pressure waves caused by respiration) に加えて、より大きな波(時には10〜40 mmHgの高さ)が見られることがあります。これらの波は、呼吸波よりもゆっくりと上昇および下降します。各サイクルの持続時間は、麻酔を受けた犬では26秒、麻酔を受けていない人間では7〜10秒です。これらの波は血管運動波 (vasomotor waves) またはマイヤー波 (Mayer waves) と呼ばれます。図18-11に示されているように、動脈圧の周期的な上昇および下降を示しています。
血管運動波の原因 (Cause of Vasomotor Waves)
血管運動波 (vasomotor waves) の原因は、1つ以上の神経性圧力制御メカニズム (nervous pressure control mechanisms) の反射振動 (reflex oscillation) によります。その一部は以下の通りです。
バロレセプターおよび化学受容器反射の振動 (Oscillation of Baroreceptor and Chemoreceptor Reflexes)
図18-11Bの血管運動波 (vasomotor waves) は、実験的な圧力記録でよく見られますが、通常は図に示されているほど強烈ではありません。これらは主にバロレセプター反射 (baroreceptor reflex) の振動によって引き起こされます。つまり、高い圧力がバロレセプター (baroreceptors) を刺激し、その結果、交感神経系が抑制され、数秒後に圧力が低下します。圧力の低下はバロレセプター刺激を減少させ、再び血管運動中枢を活性化させて圧力を高くします。この応答は瞬時には起こらず、数秒の遅れがあります。この高圧が次のサイクルを引き起こし、振動が続きます。
化学受容器反射 (chemoreceptor reflex) も同様に振動して波を引き起こすことがあります。この反射は通常、バロレセプター反射 (baroreceptor reflex) と同時に振動します。特に動脈圧が40〜80 mmHgの範囲にあるとき、化学受容器反射は主要な役割を果たします。この範囲では、化学受容器による循環制御が強力である一方、バロレセプターによる制御は弱くなります。
中枢神経系虚血反応の振動 (Oscillation of CNS Ischemic Response)
図18-11Aに示されている記録は、中枢神経系虚血圧力制御メカニズムの振動によるものです。この実験では、脳脊髄液圧 (cerebrospinal fluid pressure) が160 mmHgに増加し、脳の血管が圧迫され、中枢神経系虚血圧力反応が200 mmHgに達しました。動脈圧が非常に高い値に上昇すると、脳虚血 (brain ischemia) が緩和され、交感神経系が不活性になります。その結果、動脈圧は急速に低下し、再び脳虚血を引き起こします。その後、虚血により圧力が再び上昇します。この反応は、脳脊髄液圧が高いままである限り周期的に繰り返されます。
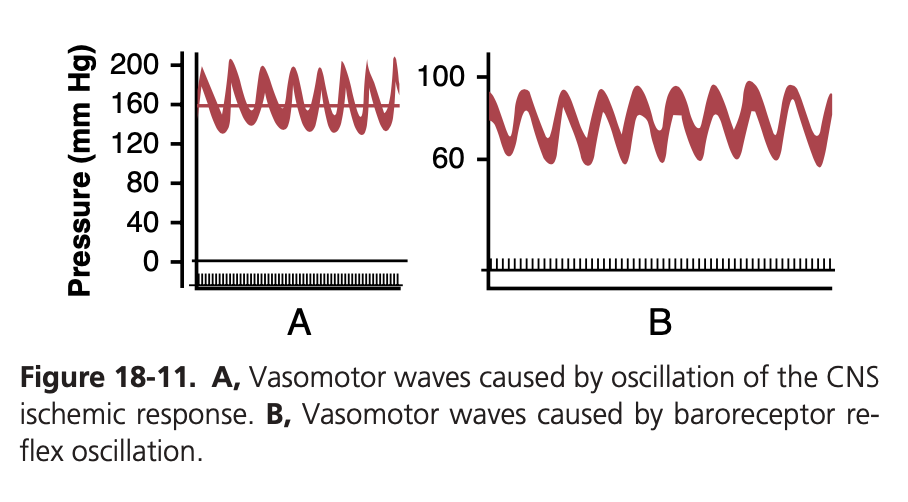
したがって、どの反射的圧力制御メカニズムでも、フィードバックの強度が十分に強く、圧力受容体 (pressure receptor) の興奮とその後の圧力応答との間に遅れがある場合、振動する可能性があります。血管運動波 (vasomotor waves) は、動脈圧を制御する神経反射が、機械的および電気的制御システムに適用される同じ原則に従うことを示しています。例えば、飛行機の自動操縦装置のガイドメカニズムにおいてフィードバック利得が強すぎ、かつ応答時間に遅れがある場合、飛行機は直進する代わりに左右に振動することになります。
NERVOUS REGULATION OF THE CIRCULATION
As discussed in Chapter 17, adjustment of blood flow in the tissues and organs of the body is mainly the function of local tissue control mechanisms. In this chapter, we discuss how nervous control of the circulation has more global functions, such as redistributing blood flow to dif- ferent areas of the body, increasing or decreasing pump- ing activity by the heart, and providing rapid control of systemic arterial pressure.
The nervous system controls the circulation almost entirely through the autonomic nervous system. The total function of this system is presented in Chapter 61, and this subject was also introduced in Chapter 17. In this chapter, we consider additional specific anatomical and functional characteristics.
AUTONOMIC NERVOUS SYSTEM
The most important part of the autonomic nervous sys- tem for regulating the circulation is the sympathetic nervous system. The parasympathetic nervous system, however, contributes importantly to regulation of heart function, as described later in the chapter.
Sympathetic Nervous System. Figure 18-1 shows the anatomy of sympathetic nervous control of the circulation. Sympathetic vasomotor nerve fibers leave the spinal cord through all the thoracic spinal nerves and through the first one or two lumbar spinal nerves. They then pass immedi- ately into a sympathetic chain, one of which lies on each side of the vertebral column. Next, they pass by two routes to the circulation: (1) through specific sympathetic nerves that innervate mainly the vasculature of the internal vis- cera and the heart, as shown on the right side of Figure 18-1; and (2) almost immediately into peripheral portions of the spinal nerves distributed to the vasculature of the peripheral areas. The precise pathways of these fibers in the spinal cord and in the sympathetic chains are discussed in Chapter 61.
Sympathetic Innervation of the Blood Vessels.
Figure 18-2 shows the distribution of sympathetic nerve fibers to the blood vessels, demonstrating that in most tis- sues, all the vessels except the capillaries are innervated. Precapillary sphincters and metarterioles are innervated in some tissues, such as the mesenteric blood vessels, although their sympathetic innervation is usually not as dense as in the small arteries, arterioles, and veins.
The innervation of the small arteries and arterioles allows sympathetic stimulation to increase resistance to blood flow and thereby decrease the rate of blood flow through the tissues.
The innervation of the large vessels, particularly of the veins, makes it possible for sympathetic stimulation to decrease the volume of these vessels. This decrease in volume can push blood into the heart and thereby plays a major role in regulation of heart pumping, as we explain later in this and subsequent chapters.
Sympathetic Stimulation Increases Heart Rate and Contractility. Sympathetic fibers also go directly to the heart, as shown in Figure 18-1. As discussed in Chapter 9, sympathetic stimulation markedly increases the activity of the heart, both increasing the heart rate and enhancing its strength and volume of pumping.
Parasympathetic Stimulation Decreases Heart Rate and Contractility. Although the parasympathetic nerv- ous system is exceedingly important for many other au- tonomic functions of the body, such as control of mul- tiple gastrointestinal actions, it plays only a minor role in regulating vascular function in most tissues. Its most important circulatory effect is to control heart rate by way of parasympathetic nerve fibers to the heart in the vagus nerves, shown in Figure 18-1 by the dashed red line from the brain medulla directly to the heart.
The effects of parasympathetic stimulation on heart function were discussed in detail in Chapter 9. Prin- cipally, parasympathetic stimulation causes a marked decrease in heart rate and a slight decrease in heart mus- cle contractility.
Sympathetic Vasoconstrictor System and Its Control by the Central Nervous System
The sympathetic nerves carry large numbers of vasocon- strictor nerve fibers and only a few vasodilator fibers. The vasoconstrictor fibers are distributed to essentially all seg- ments of the circulation, but more to some tissues than to others. This sympathetic vasoconstrictor effect is espe- cially powerful in the kidneys, intestines, spleen, and skin but is much less potent in skeletal muscle, heart, and the brain.
Vasomotor Center in the Brain and Its Control of the Vasoconstrictor System. Located bilaterally mainly in the reticular substance of the medulla and lower third of the pons is an area called the vasomotor center, shown in Figure 18-1 and Figure 18-3. This center transmits par- asympathetic impulses through the vagus nerves to the heart and sympathetic impulses through the spinal cord and peripheral sympathetic nerves to virtually all arteries, arterioles, and veins of the body.
Although the total organization of the vasomotor cen- ter is still unclear, experiments have made it possible to identify certain important areas in this center:
1. A vasoconstrictor area located bilaterally in the an- terolateral portions of the upper medulla. The neu- rons originating in this area distribute their fibers to all levels of the spinal cord, where they excite preganglionic vasoconstrictor neurons of the sym- pathetic nervous system.
- A vasodilator area located bilaterally in the ante- rolateral portions of the lower half of the medulla. The fibers from these neurons project upward to the vasoconstrictor area just described, inhibiting the vasoconstrictor activity of this area and causing vasodilation.
- A sensory area located bilaterally in the nucleus tractus solitarius in the posterolateral portions of the medulla and lower pons. The neurons of this area receive sensory nerve signals from the circu- latory system mainly through the vagus and glos- sopharyngeal nerves, and output signals from this sensory area then help control activities of the vaso- constrictor and vasodilator areas of the vasomotor center, thus providing reflex control of many circu- latory functions. An example is the baroreceptor re- flex for controlling arterial pressure, described later in this chapter.
Continuous Partial Constriction of Blood Vessels by Sympathetic Vasoconstrictor Tone. Under normal con- ditions, the vasoconstrictor area of the vasomotor center transmits signals continuously to the sympathetic vaso- constrictor nerve fibers over the entire body, causing slow firing of these fibers at a rate of about 0.5 to 2 impulses per second. This continual firing is called sympathetic vasoconstrictor tone. These impulses normally maintain a partial state of constriction in the blood vessels, called vasomotor tone.
Figure 18-4 demonstrates the significance of vaso- constrictor tone. In the experiment shown in this figure, a spinal anesthetic was administered to an animal. This anesthetic blocked all transmission of sympathetic nerve impulses from the spinal cord to the periphery. As a result, the arterial pressure fell from 100 to 50 mm Hg, demonstrating the effect of the loss of vasoconstrictor tone throughout the body. A few minutes later, a small amount of the hormone norepinephrine was injected into the blood (norepinephrine is the principal vasoconstrictor hormonal substance secreted at the endings of the sympa- thetic vasoconstrictor nerve fibers). As this injected hor- mone was transported in the blood to blood vessels, the vessels once again became constricted, and the arterial pressure rose to a level even greater than normal for 1 to 3 minutes until the norepinephrine was destroyed.
Control of Heart Activity by the Vasomotor Center.
At the same time that the vasomotor center regulates the amount of vascular constriction, it also controls heart ac- tivity. The lateral portions of the vasomotor center trans- mit excitatory impulses through the sympathetic nerve fibers to the heart when there is a need to increase heart rate and contractility. Conversely, when there is a need to decrease heart pumping, the medial portion of the vaso- motor center sends signals to the adjacent dorsal motor nuclei of the vagus nerves, which then transmit parasym- pathetic impulses through the vagus nerves to the heart to decrease heart rate and heart contractility. Therefore, the vasomotor center can increase or decrease heart activity. Heart rate and the strength of heart contractions ordinar- ily increase when vasoconstriction occurs and ordinarily decrease when vasoconstriction is inhibited.
Control of the Vasomotor Center by Higher Nervous Centers. Large numbers of small neurons located through- out the reticular substance of the pons, mesencephalon, and diencephalon can excite or inhibit the vasomotor center. This reticular substance is shown in Figure 18-3. In general, the neurons in the more lateral and superior por- tions of the reticular substance cause excitation, whereas the more medial and inferior portions cause inhibition.
The hypothalamus plays a special role in controlling the vasoconstrictor system because it can exert powerful excitatory or inhibitory effects on the vasomotor center. The posterolateral portions of the hypothalamus cause mainly excitation, whereas the anterior portion can cause mild excitation or inhibition, depending on the precise part of the anterior hypothalamus that is stimulated.
Many parts of the cerebral cortex can also excite or inhibit the vasomotor center. Stimulation of the motor cortex, for example, excites the vasomotor center because of impulses transmitted downward into the hypothala- mus and then to the vasomotor center. Also, stimulation of the anterior temporal lobe, orbital areas of the frontal cortex, anterior part of the cingulate gyrus, amygdala, sep- tum, and hippocampus can all excite or inhibit the vaso- motor center, depending on the precise portions of these areas that are stimulated and the intensity of the stimulus. Thus, widespread basal areas of the brain can have pro- found effects on cardiovascular function.
Norepinephrine Is the Sympathetic Vasoconstrictor Neurotransmitter. The substance secreted at the endings of the vasoconstrictor nerves is almost entirely norepi- nephrine, which acts directly on the alpha-adrenergic receptors of the vascular smooth muscle to cause vaso- constriction, as discussed in Chapter 61.
Adrenal Medullae and Their Relationship to the Sym- pathetic Vasoconstrictor System. Sympathetic impulses are transmitted to the adrenal medullae at the same time that they are transmitted to the blood vessels. These im- pulses cause the medullae to secrete epinephrine and nor- epinephrine into the circulating blood. These two hormones are carried in the blood stream to all parts of the body, where they act directly on all blood vessels and usually cause vasoconstriction. In a few tissues, epinephrine causes vasodilation because it also stimulates beta-adrenergic re- ceptors, which dilates rather than constricts certain vessels, as discussed in Chapter 61.
Sympathetic Vasodilator System and Its Control by the Central Nervous System. The sympathetic nerves to skel- etal muscles carry sympathetic vasodilator fibers, as well as constrictor fibers. In some animals, such as the cat, these dilator fibers release acetylcholine, not norepinephrine, at their endings. However, in primates, the vasodilator effect is believed to be caused by epinephrine exciting specific beta-adrenergic receptors in the muscle vasculature.
The pathway for central nervous system (CNS) control of the vasodilator system is shown by the dashed lines in Figure 18-3. The principal area of the brain controlling this system is the anterior hypothalamus.
Possible Role of the Sympathetic Vasodilator System.
The sympathetic vasodilator system does not appear to play a major role in the control of the circulation in humans be- cause complete block of the sympathetic nerves to the mus- cles hardly affects the ability of these muscles to control their own blood flow in many physiological conditions. Yet, some experiments have suggested that at the onset of exercise, the sympathetic system might cause initial vasodilation in skel- etal muscles to allow an anticipatory increase in blood flow, even before the muscles require increased nutrients. There is evidence in humans that this sympathetic vasodilator re- sponse in skeletal muscles may be mediated by circulating epinephrine, which stimulates beta-adrenergic receptors, or by nitric oxide released from the vascular endothelium in re- sponse to stimulation by acetylcholine.
Emotional Fainting—Vasovagal Syncope. An interest- ing vasodilatory reaction occurs in people who experience intense emotional disturbances that cause fainting. In this case, the muscle vasodilator system becomes activated and, at the same time, the vagal cardioinhibitory center transmits strong signals to the heart to slow the heart rate markedly. The arterial pressure falls rapidly, which reduces blood flow to the brain and causes the person to lose con- sciousness. This overall effect is called vasovagal syncope. Emotional fainting begins with disturbing thoughts in the cerebral cortex. The pathway probably then goes to the vas- odilatory center of the anterior hypothalamus next to the vagal centers of the medulla, to the heart through the vagus nerves, and also through the spinal cord to the sympathetic vasodilator nerves of the muscles.
Role of the Nervous System in Rapid Control of Arterial Pressure
One of the most important functions of nervous control of the circulation is its capability to cause rapid increases in arterial pressure. For this purpose, the entire vaso- constrictor and cardioaccelerator functions of the sym- pathetic nervous system are stimulated together. At the same time, there is reciprocal inhibition of parasympa- thetic vagal inhibitory signals to the heart. Thus, the fol- lowing three major changes occur simultaneously, each of which helps increase arterial pressure:
1. Most arterioles of the systemic circulation are con- stricted, which greatly increases the total peripheral resistance, thereby increasing the arterial pressure.
2. The veins especially (but the other large vessels of the circulation as well) are strongly constricted. This constriction displaces blood out of the large periph- eral blood vessels toward the heart, thus increasing the volume of blood in the heart chambers. The stretch of the heart then causes the heart to beat with greater force and therefore to pump increased quantities of blood. This also increases the arterial pressure.
3. Finally, the heart is directly stimulated by the au- tonomic nervous system, further enhancing cardiac pumping. Much of this enhanced cardiac pumping is caused by an increase in the heart rate, which some- times increases to as much as three times normal. In addition, sympathetic nervous signals directly increase the contractile force of the heart muscle, increasing the capability of the heart to pump larger volumes of blood. During strong sympathetic stimu- lation, the heart can pump about two times as much blood as under normal conditions, which contrib- utes still more to the acute rise in arterial pressure.
Nervous Control of Arterial Pressure Is Rapid. An es- pecially important characteristic of nervous control of ar- terial pressure is its rapidity of response, beginning within seconds and often increasing the pressure to two times normal within 5 to 10 seconds. Conversely, sudden inhi- bition of nervous cardiovascular stimulation can decrease the arterial pressure to as little as half-normal within 10 to 40 seconds. Therefore, nervous control is the most rapid mechanism for arterial pressure regulation.
INCREASES IN ARTERIAL PRESSURE DURING MUSCLE EXERCISE AND OTHER STRESSES
An important example of the nervous system’s ability to increase arterial pressure is the rise in pressure that occurs during muscle exercise. During heavy exercise, the muscles require greatly increased blood flow. Part of this increase results from local vasodilation of the muscle vas- culature caused by increased metabolism of the muscle cells, as explained in Chapter 17. An additional increase results from simultaneous elevation of arterial pressure caused by sympathetic stimulation of the overall circula- tion during exercise. In heavy exercise, the arterial pres- sure rises by about 30% to 40%, which further increases blood flow by almost 2-fold.
The increase in arterial pressure during exercise results mainly from effects of the nervous system. At the same time that the motor areas of the brain become activated to cause exercise, most of the reticular activating system of the brain stem is also activated, which includes greatly increased stimulation of the vasoconstrictor and cardio- acceleratory areas of the vasomotor center. These effects rapidly increase the arterial pressure to keep pace with the increase in muscle activity.
In many other types of stress besides muscle exercise, a similar rise in pressure can also occur. For example, dur- ing extreme fright, the arterial pressure sometimes rises by as much as 75 to 100 mm Hg within a few seconds. This response is called the alarm reaction, and it provides an elevated arterial pressure that can immediately supply blood to the muscles of the body that might be needed to respond instantly to enable flight from danger.
REFLEX MECHANISMS FOR MAINTAINING NORMAL ARTERIAL PRESSURE
Aside from the exercise and stress functions of the auto- nomic nervous system to increase arterial pressure, multiple subconscious special nervous control mecha- nisms operate all the time to maintain the arterial pressure at or near normal. Almost all these are negative feedback reflex mechanisms, described in the following sections.
Baroreceptor Arterial Pressure Control System—Baroreceptor Reflexes
The best known of the nervous mechanisms for arterial pressure control is the baroreceptor reflex. Basically, this reflex is initiated by stretch receptors, called baroreceptors or pressoreceptors, located at specific points in the walls of several large systemic arteries. A rise in arterial pressure stretches the baroreceptors and causes them to transmit signals into the CNS. Feedback signals are then sent back through the autonomic nervous system to the circulation to reduce arterial pressure down toward the normal level.
Physiologic Anatomy of the Baroreceptors and Their Innervation. Baroreceptors are spray-type nerve end- ings that lie in the walls of the arteries and are stimulated when stretched. A few baroreceptors are located in the wall of almost every large artery of the thoracic and neck regions but, as shown in Figure 18-5, baroreceptors are extremely abundant in the following regions: (1) the wall of each internal carotid artery, slightly above the carotid bifurcation, an area known as the carotid sinus; and (2) the wall of the aortic arch.
Figure 18-5 shows that signals from the carotid baro- receptors are transmitted through small Hering’s nerves to the glossopharyngeal nerves in the high neck and then to the nucleus tractus solitarius in the medullary area of the brain stem. Signals from the aortic baroreceptors in the arch of the aorta are transmitted through the vagus nerves to the same nucleus tractus solitarius of the medulla.
Response of the Baroreceptors to Changes in Arte- rial Pressure. Figure 18-6 shows the effects of different arterial pressure levels on the rate of impulse transmission in a Hering’s carotid sinus nerve. Note that the carotid sinus baroreceptors are not stimulated at all by pressures between 0 and 50 to 60 mm Hg but, above these levels, they respond progressively more rapidly and reach a max- imum at about 180 mm Hg. The responses of the aortic baroreceptors are similar to those of the carotid receptors except that they operate, in general, at arterial pressure levels about 30 mm Hg higher.
Note especially that in the normal operating range of arterial pressure, around 100 mm Hg, even a slight change in pressure causes a strong change in the baroreflex signal to readjust arterial pressure back toward normal. Thus, the baroreceptor feedback mechanism functions most effectively in the pressure range where it is most needed.
The baroreceptors respond rapidly to changes in arte- rial pressure; the rate of impulse firing increases in the fraction of a second during each systole and decreases again during diastole. Furthermore, the baroreceptors respond much more to a rapidly changing pressure than to a stationary pressure. That is, if the mean arterial pressure is 150 mm Hg but at that moment is rising rapidly, the rate of impulse transmission may be as much as twice that when the pressure is stationary at 150 mm Hg.
Circulatory Reflex Initiated by the Baroreceptors.
After the baroreceptor signals have entered the nucleus tractus solitarius of the medulla, secondary signals inhibit the vasoconstrictor center of the medulla and excite the va- gal parasympathetic center. The net effects are as follows: (1) vasodilation of the veins and arterioles throughout the peripheral circulatory system; and (2) decreased heart rate and strength of heart contraction. Therefore, excita- tion of the baroreceptors by high pressure in the arteries reflexly causes the arterial pressure to decrease because of a decrease in peripheral resistance and a decrease in cardiac output. Conversely, low pressure has the opposite effects, reflexly causing the pressure to rise back toward normal.
Figure 18-7 shows a typical reflex change in arterial pressure caused by occluding the two common carotid arteries. This reduces the carotid sinus pressure; as a result, signals from the baroreceptors decrease and cause less inhibitory effect on the vasomotor center. The vasomotor center then becomes much more active than usual, causing the aortic arterial pressure to rise and remain elevated during the 10 minutes that the carot- ids are occluded. Removal of the occlusion allows the pressure in the carotid sinuses to rise, and the carotid sinus reflex now causes the aortic pressure to fall almost immediately to slightly below normal as a momentary overcompensation and then return to normal in another minute.
Baroreceptors Attenuate Blood Pressure Changes During Changes in Body Posture. The ability of the ba- roreceptors to maintain relatively constant arterial pres- sure in the upper body is important when a person stands up after lying down. Immediately on standing, the arterial pressure in the head and upper part of the body tends to fall, and marked reduction of this pressure could cause loss of consciousness. However, the falling pressure at the baroreceptors elicits an immediate reflex, resulting in strong sympathetic discharge throughout the body that minimizes the decrease in pressure in the head and up- per body.
Pressure Buffer Function of the Baroreceptor Control System. Because the baroreceptor system opposes in- creases or decreases in arterial pressure, it is called a pres- sure buffer system, and the nerves from the baroreceptors are called buffer nerves.
Figure 18-8 shows the importance of this buffer func- tion of the baroreceptors. The upper panel in this figure shows an arterial pressure recording for 2 hours from a normal dog, and the lower panel shows an arterial pres- sure recording from a dog whose baroreceptor nerves from the carotid sinuses and the aorta had been removed. Note the extreme variability of pressure in the denervated dog caused by simple events of the day, such as lying down, standing, excitement, eating, defecation, and noises.
Figure 18-9 shows the frequency distributions of the mean arterial pressures recorded for a 24-hour day in the normal dog and the denervated dog. Note that when the baroreceptors were functioning normally, the mean arte- rial pressure remained within a narrow range of between 85 and 115 mm Hg throughout the day and, for most of the day, it remained at about 100 mm Hg. After denerva- tion of the baroreceptors, however, the frequency distri- bution curve flattened, showing that the pressure range increased 2.5-fold, frequently falling to as low as 50 mm Hg or rising to more than 160 mm Hg. Thus, one can see the extreme variability of pressure in the absence of the arterial baroreceptor system.
A primary purpose of the arterial baroreceptor system is therefore to reduce the minute by minute variation in arterial pressure to about one-third that which would occur if the baroreceptor system were not present.
Are the Baroreceptors Important in Long-Term Regulation of Arterial Pressure? Although the arte- rial baroreceptors provide powerful moment to moment control of arterial pressure, their importance in long-term blood pressure regulation has been controversial. One rea- son that the baroreceptors have been considered by some physiologists to be relatively unimportant in chronic regula- tion of arterial pressure is that they tend to reset in 1 to 2 days to the pressure level to which they are exposed. That is, if the arterial pressure rises from the normal value of 100 to 160 mm Hg, a very high rate of baroreceptor impulses is at first transmitted. During the next few minutes, the rate of firing diminishes considerably. Then, it diminishes much more slowly during the next 1 to 2 days, at the end of which time the rate of firing will have returned to nearly normal, despite the fact that the mean arterial pressure still remains at 160 mm Hg. Conversely, when the arterial pressure falls to a very low level, the baroreceptors at first transmit no im- pulses but gradually, over 1 to 2 days, the rate of barorecep- tor firing returns toward the control level.
This resetting of the baroreceptors may attenuate their potency as a control system for correcting disturbances that tend to change arterial pressure for longer than a few days at a time. Experimental studies, however, have sug- gested that the baroreceptors do not completely reset and may therefore contribute to long-term blood pressure reg- ulation, especially by influencing sympathetic nerve activ- ity of the kidneys. For example, with prolonged increases in arterial pressure, the baroreceptor reflexes may mediate decreases in renal sympathetic nerve activity that promote increased excretion of sodium and water by the kidneys. This action, in turn, causes a gradual decrease in blood volume, which helps restore arterial pressure toward nor- mal. Thus, long-term regulation of mean arterial pressure by the baroreceptors requires interaction with additional systems, principally the renal–body fluid–pressure control system (along with its associated nervous and hormonal mechanisms), discussed in Chapters 19 and 30.
Experimental studies and clinical trials have shown that chronic electrical stimulation of carotid sinus afferent nerve fibers can cause sustained reductions in sympathetic nervous system activity and arterial pressure of at least 15 to 20 mm Hg. These observations suggest that most, if not all, the baroreceptor reflex resetting that occurs when increases in arterial pressure are sustained, as in chronic hypertension, is due to resetting of the carotid sinus nerve mechanoreceptors themselves rather than resetting in central nervous system vasomotor centers.
Control of Arterial Pressure by the Carotid and Aortic Chemoreceptors—Effect of Low Oxygen on Arterial Pressure. Closely associated with the baroreceptor pres- sure control system is a chemoreceptor reflex that operates in much the same way as the baroreceptor reflex except that chemoreceptors, instead of stretch receptors, initiate the response.
The chemoreceptor cells are sensitive to low oxygen or elevated carbon dioxide and hydrogen ion levels. They are located in several small chemoreceptor organs about 2 millimeters in size (two carotid bodies, one of which lies in the bifurcation of each common carotid artery, and usually one to three aortic bodies adjacent to the aorta). The chemoreceptors excite nerve fibers that along with the baroreceptor fibers, pass through Hering’s nerves and the vagus nerves into the vasomotor center of the brain stem.
Each carotid or aortic body is supplied with an abun- dant blood flow through a small nutrient artery, so the chemoreceptors are always in close contact with arterial blood. Whenever the arterial pressure falls below a criti- cal level, the chemoreceptors become stimulated because diminished blood flow causes decreased oxygen, as well as excess buildup of carbon dioxide and hydrogen ions that are not removed by the slowly flowing blood.
The signals transmitted from the chemoreceptors excite the vasomotor center, and this response elevates the arte- rial pressure back toward normal. However, this chemore- ceptor reflex is not a powerful arterial pressure controller until the arterial pressure falls below 80 mm Hg. Therefore, it is at the lower pressures that this reflex becomes impor- tant to help prevent further decreases in arterial pressure.
The chemoreceptors are discussed in much more detail in Chapter 42 in relation to respiratory control, in which they normally play a far more important role than in blood pressure control. However, activation of the chemorecep- tors may also contribute to increases in arterial pressure in conditions such as severe obesity and obstructive sleep apnea, a serious sleep disorder associated with repetitive episodes of nocturnal breathing cessation and hypoxia.
Atrial and Pulmonary Artery Reflexes Regulate Arteri- al Pressure. The atria and pulmonary arteries have stretch receptors in their walls called low-pressure receptors. Low- pressure receptors are similar to the baroreceptor stretch receptors of the large systemic arteries. These low-pressure receptors play an important role, especially in minimizing arterial pressure changes in response to changes in blood volume. For example, if 300 milliliters of blood suddenly are infused into a dog with all receptors intact, the arte- rial pressure rises only about 15 mm Hg. With the arterial baroreceptors denervated, the pressure rises about 40 mm Hg. If the low-pressure receptors also are denervated, the arterial pressure rises about 100 mm Hg.
Thus, one can see that even though the low-pressure receptors in the pulmonary artery and in the atria cannot detect the systemic arterial pressure, they do detect simul- taneous increases in pressure in the low-pressure areas of the circulation caused by increase in volume. Also, they elicit reflexes parallel to the baroreceptor reflexes to make the total reflex system more potent for control of arterial pressure.
Atrial Reflexes That Activate the Kidneys—The Volume Reflex. Stretch of the atria and activation of low-pressure atrial receptors also causes reflex reductions in renal sympathetic nerve activity, decreased tubular re- absorption, and dilation of afferent arterioles in the kid- neys (Figure 18-10). Signals are also transmitted simul- taneously from the atria to the hypothalamus to decrease secretion of antidiuretic hormone (ADH). The decreased afferent arteriolar resistance in the kidneys causes the glomerular capillary pressure to rise, with a resultant in- crease in filtration of fluid into the kidney tubules. The de- crease in ADH level diminishes the reabsorption of water from the tubules. The combination of these effects—an increase in glomerular filtration and a decrease in reab- sorption of the fluid—increases fluid loss by the kidneys and attenuates the increased blood volume. Atrial stretch caused by increased blood volume also elicits release of atrial natriuretic peptide, a hormone that adds further to the excretion of sodium and water in the urine and return of blood volume toward normal (see Figure 18-10).
All these mechanisms that tend to return blood volume back toward normal after a volume overload act indirectly as pressure controllers, as well as blood volume control- lers, because excess volume drives the heart to greater cardiac output and higher arterial pressure. This volume reflex mechanism is discussed again in Chapter 30, along with other mechanisms of blood volume control.
Increased Atrial Pressure Raises Heart Rate—Bainbridge Reflex. Increases in atrial pressure sometimes increase the heart rate as much as 75%, particularly when the prevailing heart rate is slow. When the heart rate is rapid, atrial stretch cause by infusion of fluids may reduce the heart rate due to activation of arterial baroreceptors. Thus, the net effect of increased blood volume and atrial stretch on heart rate de- pends on the relative contributions of the baroreceptor re- flexes (which tends to slow the heart rate) and the Bainbridge reflex which tends to accelerate the heart rate, as shown in Figure 18-10. When blood volume is increased above nor- mal, the Bainbridge reflex often increases heart rate despite the inhibitory actions of the baroreflexes.
A small part of the increased heart rate associated with increased blood volume and atrial stretch is caused by a direct effect of the increased atrial volume to stretch the sinus node; it was noted in Chapter 10 that such direct stretch can increase the heart rate as much as 15%. An additional 40% to 60% increase in heart rate is caused by the Bainbridge reflex. The stretch receptors of the atria that elicit the Bainbridge reflex transmit their afferent sig- nals through the vagus nerves to the medulla of the brain. Then efferent signals are transmitted back through vagal and sympathetic nerves to increase the heart rate and strength of heart contraction. Thus, this reflex helps pre- vent damming of blood in the veins, atria, and pulmonary circulation.
DECREASED BLOOD FLOW TO BRAIN VASOMOTOR CENTER ELICITS INCREASED BLOOD PRESSURE—CNS ISCHEMIC RESPONSE
Most nervous control of blood pressure is achieved by reflexes that originate in the baroreceptors, chemoreceptors, and low-pressure receptors, all of which are located in the peripheral circulation outside the brain. However, when blood flow to the vasomotor center in the lower brain stem becomes decreased severely enough to cause nutri- tional deficiency—that is, to cause cerebral ischemia—the vasoconstrictor and cardioaccelerator neurons in the vasomotor center respond directly to the ischemia and become strongly excited. When this excitation occurs, the systemic arterial pressure often rises to a level as high as the heart can possibly pump. This effect is believed to be caused by failure of the slowly flowing blood to carry car- bon dioxide away from the brain stem vasomotor center. At low levels of blood flow to the vasomotor center, the local concentration of carbon dioxide increases greatly and has an extremely potent effect in stimulating the sym- pathetic vasomotor nervous control areas in the brain’s medulla.
It is possible that other factors, such as buildup of lactic acid and other acidic substances in the vasomotor center, also contribute to the marked stimulation and elevation in arterial pressure. This arterial pressure elevation in response to cerebral ischemia is known as the CNS isch- emic response.
The ischemic effect on vasomotor activity can elevate the mean arterial pressure dramatically, sometimes to as high as 250 mm Hg for as long as 10 minutes. The degree of sympathetic vasoconstriction caused by intense cerebral ischemia is often so great that some of the peripheral ves- sels become totally or almost totally occluded. The kidneys, for example, often cease their production of urine entirely because of renal arteriolar constriction in response to the sympathetic discharge. Therefore, the CNS ischemic response is one of the most powerful of all the activators of the sympathetic vasoconstrictor system.
Importance of CNS Ischemic Response as a Regulator of Arterial Pressure. Despite the powerful nature of the CNS ischemic response, it does not become significant until the arterial pressure falls far below normal, down to 60 mm Hg and below, reaching its greatest degree of stimulation at a pressure of 15 to 20 mm Hg. Therefore, the CNS ischemic response is not one of the normal mechanisms for regulating arterial pressure. Instead, it operates principally as an emergency pressure control sys- tem that acts rapidly and powerfully to prevent further decrease in arterial pressure whenever blood flow to the brain decreases dangerously close to the lethal level. It is sometimes called the last-ditch stand pressure control mechanism.
Cushing Reaction to Increased Pressure Around the Brain. The Cushing reaction is a special type of CNS is- chemic response that results from increased pressure of the cerebrospinal fluid around the brain in the cranial vault. For example, when the cerebrospinal fluid pres- sure rises to equal the arterial pressure, it compresses the whole brain, as well as the arteries in the brain, and cuts off the blood supply to the brain. This action initiates a CNS ischemic response that causes the arterial pressure to rise. When the arterial pressure has risen to a level higher than the cerebrospinal fluid pressure, blood will flow once again into the vessels of the brain to relieve the brain ischemia. Ordinarily, the blood pressure reaches a new equilibrium level slightly higher than the cerebrospi- nal fluid pressure, thus allowing blood to begin to flow through the brain again. The Cushing reaction helps pro- tect vital centers of the brain from loss of nutrition if the cerebrospinal fluid pressure ever rises high enough to compress the cerebral arteries.
SPECIAL FEATURES OF NERVOUS CONTROL OF ARTERIAL PRESSURE
ROLE OF THE SKELETAL NERVES AND SKELETAL MUSCLES IN INCREASING CARDIAC OUTPUT AND ARTERIAL PRESSURE
Although most rapidly acting nervous control of the circu- lation is affected through the autonomic nervous system, at least two conditions exist in which the skeletal nerves and muscles also play major roles in circulatory responses.
Abdominal Compression Reflex Increases Cardiac Output and Arterial Pressure. When a baroreceptor or chemoreceptor reflex is elicited, nerve signals are transmitted simultaneously through skeletal nerves to skeletal muscles of the body, particularly to the abdomi- nal muscles. Muscle contraction then compresses all the venous reservoirs of the abdomen, helping translocate blood out of the abdominal vascular reservoirs toward the heart. As a result, increased quantities of blood are made available for the heart to pump. This overall re- sponse is called the abdominal compression reflex. The resulting effect on the circulation is the same as that caused by sympathetic vasoconstrictor impulses when they constrict the veins—an increase in both cardiac output and arterial pressure. The abdominal compres- sion reflex is probably much more important than was realized in the past because it is well known that people whose skeletal muscles have been paralyzed are consid- erably more prone to hypotensive episodes than people with normal skeletal muscles.
Skeletal Muscle Contraction Increases Cardiac Output and Arterial Pressure During Exercise. When the skeletal muscles contract during exercise, they compress blood vessels throughout the body. Even anticipation of exercise tightens the muscles, thereby compressing the vessels in the muscles and in the ab- domen. This compression translocates blood from the peripheral vessels into the heart and lungs and, there- fore, increases cardiac output. This effect is essential in helping cause the fivefold to sevenfold increase in cardiac output that sometimes occurs during heavy ex- ercise. The rise in cardiac output, in turn, is an essen- tial ingredient in increasing the arterial pressure during exercise, from a normal mean of 100 mm Hg up to 130 to 160 mm Hg.
RESPIRATORY WAVES IN THE ARTERIAL PRESSURE
With each cycle of respiration, the arterial pressure usu- ally rises and falls 4 to 6 mm Hg in a wavelike manner, causing respiratory waves in the arterial pressure. The waves result from several different effects, some of which are reflex in nature, as follows:
1. Many of the breathing signals that arise in the res- piratory center of the medulla spill over into the vasomotor center with each respiratory cycle.
2. Every time a person inspires, the pressure in the thoracic cavity becomes more negative than usual, causing the blood vessels in the chest to expand. This reduces the quantity of blood returning to the left side of the heart and thereby momentarily de- creases the cardiac output and arterial pressure.
3. The pressure changes caused in the thoracic vessels by respiration can excite vascular and atrial stretch receptors.
Although it is difficult to analyze the exact relations of all these factors in causing the respiratory pressure waves, the net result during normal respiration is usually an increase in arterial pressure during the early part of expi- ration and a decrease in pressure during the remainder of the respiratory cycle. During deep respiration, the blood pressure can rise and fall as much as 20 mm Hg with each respiratory cycle.
Arterial Pressure Vasomotor Waves— Oscillation of Pressure Reflex Control Systems
Often while recording arterial pressure, in addition to the small pressure waves caused by respiration, some much larger waves are also noted—as high as 10 to 40 mm Hg at times—that rise and fall more slowly than the respi- ratory waves. The duration of each cycle varies from 26 seconds in the anesthetized dog to 7 to 10 seconds in the unanesthetized human. These waves are called vasomo- tor waves or Mayer waves. Such records are illustrated in Figure 18-11, showing the cyclical rise and fall in arterial pressure.
The cause of vasomotor waves is reflex oscillation of one or more nervous pressure control mechanisms, some of which are the following.
Oscillation of Baroreceptor and Chemoreceptor Reflexes. The vasomotor waves of Figure 18-11B are often seen in experimental pressure recordings, al- though they are usually much less intense than shown in the figure. They are caused mainly by oscillation of the baroreceptor reflex. That is, a high pressure excites the baroreceptors, which then inhibits the sympathetic nervous system and lowers the pressure a few seconds later. The decreased pressure, in turn, reduces the ba- roreceptor stimulation and allows the vasomotor cent- er to become active once again, elevating the pressure to a high value. The response is not instantaneous, and it is delayed until a few seconds later. This high pres- sure then initiates another cycle, and the oscillation continues.
The chemoreceptor reflex can also oscillate to give the same type of waves. This reflex usually oscillates simul- taneously with the baroreceptor reflex. It probably plays the major role in causing vasomotor waves when the arte- rial pressure is in the range of 40 to 80 mm Hg because, in this low range, chemoreceptor control of the circula- tion becomes powerful, whereas baroreceptor control becomes weaker.
Oscillation of CNS Ischemic Response. The record in Figure 18-11A resulted from oscillation of the CNS is- chemic pressure control mechanism. In this experiment, the cerebrospinal fluid pressure increased to 160 mm Hg, which compressed the cerebral vessels and initiated a CNS ischemic pressure response up to 200 mm Hg. When the arterial pressure rose to such a high value, the brain ischemia was relieved, and the sympathetic nervous system became inactive. As a result, the arterial pressure fell rapidly back to a much lower value, causing brain is- chemia once again. The ischemia then initiated another rise in pressure. Again, the ischemia was relieved, and again the pressure fell. This response repeated itself cyclically as long as the cerebrospinal fluid pressure remained elevated.
Thus, any reflex pressure control mechanism can oscil- late if the intensity of feedback is strong enough, and if there is a delay between excitation of the pressure receptor and the subsequent pressure response. The vasomotor waves illus- trate that the nervous reflexes that control arterial pressure obey the same principles as those applicable to mechanical and electrical control systems. For example, if the feedback gain is too great in the guiding mechanism of an automatic pilot for an airplane, and there is also delay in the response time of the guiding mechanism, the plane will oscillate from side to side instead of following a straight course.
コメント